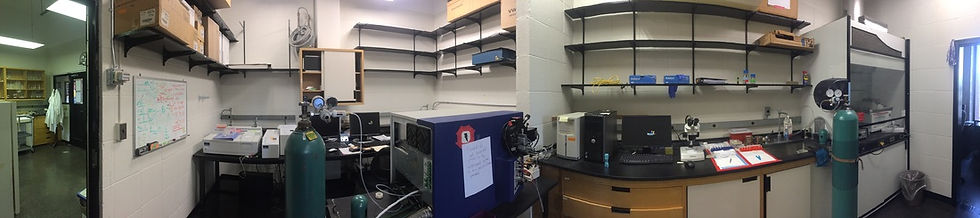
Research
Research Projects
Experimental Hydrogen Exchange Mass Spectrometry (HXMS)
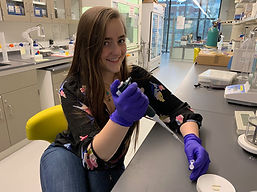
We combine experimental and computational techniques to extend ongoing work using HXMS to investigate the folding landscapes of proteins in order to understand the relationship between protein function and dynamics. Proteins being studied include beta-2 microglobulin, protein L, trypsinogen, beta-lactamases and myoglobin.
Numerical simulations to fit HXMS experimental results
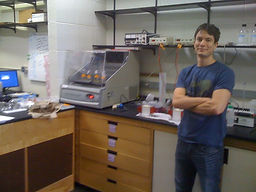
This computational project will build on ongoing work using Bayesian statistics to model protein folding dynamics and hydrogen exchange behavior for intact proteins, and for protein fragments.
Validation of Acetonitrile as a Protein Denaturant
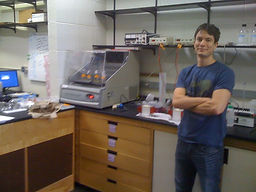
Biochemists have typically added high concentrations of denaturants such as urea or guanidine in order to unfold proteins and measure their energetic landscapes. Unfortunately, neither of these denaturants is compatible with HXMS but a less commonly used denaturant, acetonitrile is. How similar are the mechanisms of acetonitrile protein denaturation to those of urea and guanidine?
Why do we study proteins?
Proteins are the molecules that carry out the vast majority of the jobs necessary to keep cells functioning, including breaking down and synthesizing nutrients, carrying messages and cargo, providing structural support, and raising the alarm and defending against attack. The code by which sets of three nucleotides in DNA specify the 20 chemical building blocks known as amino acids that comprise the alphabet of proteins was deciphered long ago. With the explosion of genome sequencing, the order in which amino acids need to be strung together to make each and every protein that is found in nearly 200 different organisms, including human, is now known. There is tremendous potential within this wealth of sequence information to contribute to better understanding of biology and to improve medicine by pinpointing differences in proteins from different samples – for example between healthy and tumor cells. Despite recent advances in protein structural determination such as AlphaFold, understanding the folding and dynamics of proteins, critical for protein function, remains an open problem.
The Missing Link
How is protein sequence linked to biological function? This missing link arises because proteins function not as linear chains of amino acids, but rather each protein folds into a specific compact three-dimensional structure, whose shape and dynamics are the key to its ability to carry out its particular tasks. Cracking the code for this molecular origami – how an amino acid sequence specifies the correctly folded structure, the pathway for reaching it, and the ability to remain stable and folded – is the fundamental puzzle that has captivated protein chemists for decades. Because some proteins spontaneously find their way back to the same folded structure after being unfolded in the test-tube, investigators have found clues into the folding process by “interrogating” proteins through heating or adding chemicals to the protein sample, and watching them unfold, then refold when returned to less harsh conditions. While the harsh conditions of traditional folding methods yield insight into folding for model proteins that are “well-behaved” and resilient to being harassed by heat and chemicals, most proteins do not recover and little information about their folding is gained through such treatment.
What are we doing?
Our lab studies protein folding using an array of biophysical, biochemical, and computational approaches. We focus on developing techniques to allow “folding-challenged” proteins that are very different from most model proteins to be studied. These rogue proteins may have an extremely high energy barrier that prevents the folded structure from unfolding and/or additional metastable conformations accessible in their folding landscapes that become stable upon aggregation. We are developing a milder technique to probe folding that exploits mass spectrometry to expand the range of proteins accessible to folding investigations. Since protein misfolding has been linked to an ever-increasing number of diseases – including neurodegeneration, aging, cancer, and HIV infection – over the past two decades, the ability to explore the full diversity of mechanisms for coupling protein folding to biological function will be crucial for understanding the pathology of these diseases as well as for developing novel design strategies for therapeutic and engineering purposes.